Very. As far as we know, the Higgs boson is only second in mass to the top quark – that remains at, well, the top of the weighing scale for known elementary particles. And even if it is a silver medalist, the Higgs boson packs a punch, weighing in at 125 GeV – give or take some 0.3 GeV. But what is also massive is the plethora of results about this Higgs boson that the CMS Collaboration has produced since the particle's discovery in 2012.
The recent CMS submission to the European Physical Journal C, available as arXiv:1412.8662, brings together the broadest set of results on the Higgs boson and its properties to date. This work marks, to a large extent, the end of a chapter in the story of this particle, namely what we have learnt about the Higgs boson from the LHC Run 1 data. With the LHC poised to start Run 2 in 2015, this paper was fittingly submitted on 30 December 2014 and with another CMS paper on the spin and parity of the boson, arXiv:1411.3441, it draws a picture that – for the moment – cannot be distinguished from the Standard Model (SM) predictions for the Higgs boson.
The mass of the SM Higgs boson is one of the fundamental SM parameters. Two channels – one in which the Higgs decays into two photons and the other in which it decays to two Z bosons and subsequently four leptons – provide the best mass resolution. The combined analysis of these decay channels allows CMS to extract the most precise measurement to date: 125.02±0.30 GeV, a relative uncertainty of 0.2%. Such precision is the result of a very big effort put into understanding the CMS detector: crucially the calibration of the ECAL used to observe electrons and photons and of the muon tracking detectors. The uncertainty can be split into a systematic component (±0.15 GeV) and a statistical component (±0.26 GeV). This provides excellent prospects for Run 2 to yield an even more precise mass measurement, as the statistical component can be reduced by the accumulation of more data.
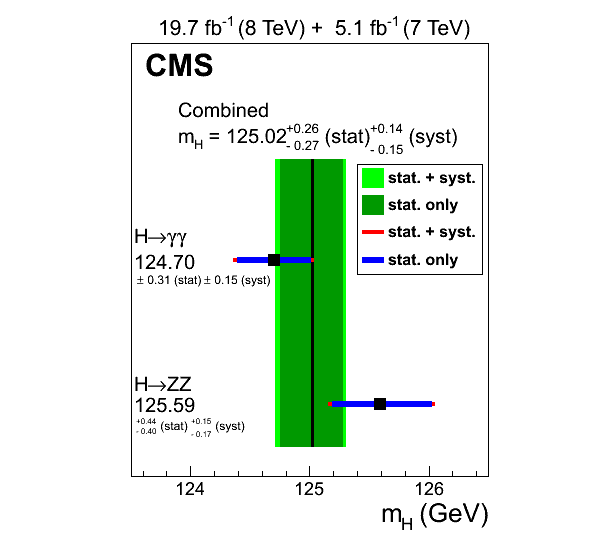
The SM has been a remarkable framework; coupled to increasingly-more-precise measurements of mesons and electroweak bosons, it has been able since the late 1980s to predict the mass of the top quark and later the mass of the Higgs boson. With the discovery of the Higgs boson, the SM became complete: for the first time we have experimental measurements of all SM parameters, the last such parameter being the mass of the Higgs boson. This does not mean that we are finished with SM, but quite the opposite. With all parameters experimentally constrained we can now use the SM to make even more accurate predictions. For instance, with the existing measurements, the SM now predicts the mass of the W boson with a precision almost twice as good as that of the experimental measurements.
As far as we can tell, the SM provides the most reliable baseline description of reality; we search for deviations or excesses with respect to this baseline as hints pointing to new beyond-the-SM (BSM) physics. Having now measured the mass of the Higgs boson, the SM makes unambiguous predictions as to what the Higgs boson's other properties should be. Some, such as the boson's spin (zero), parity (positive), and electric charge (neutral) stem directly from the symmetries of the SM. But others, such as the strength with which the Higgs boson interacts (or couples) with other SM particles are not integers. Assuming that there is only one particle being observed, the consistency between the observations in decays to bosons and fermions is checked against the SM predictions.
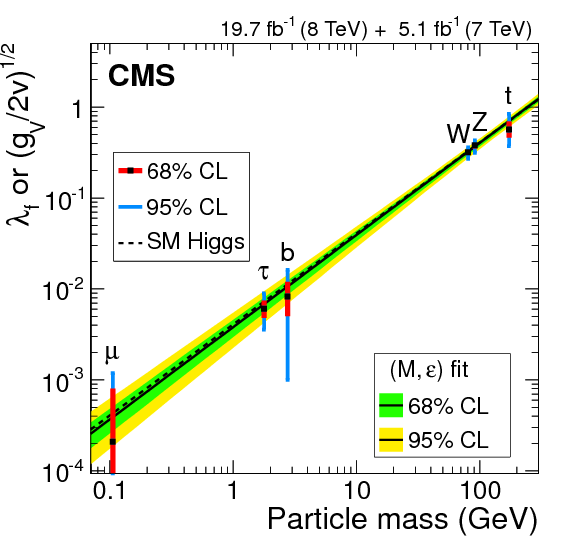
The set of measurements that is analysed includes the decays of the Higgs boson to photons, Z bosons, W bosons, tau leptons, b quarks and muons, and includes specialised studies targeting the different production modes predicted by the SM, where the Higgs boson is produced in association with at least one other particle. Finally, analyses searching for invisible decays of the Higgs boson (presumably into BSM particles) allow us to complete the picture by constraining what the total width of the Higgs boson may be.
Much could be written about the hundreds of thousand of CPU hours spent computing the likelihood values used to perform the measurements, much more could be written about the exact values and uncertainties extracted, and even more on how the work of thousands of people during of decades can be distilled in a file that is about 1 MB in size. But the essence is that up to now "no significant deviations from the SM predictions were found".
Finding no significant deviations sets the bar high for Run 2. We will have to be smarter as the LHC data pour in and push us beyond the amount of information available from Run 1. Both theorists and experimentalists will continue working together to find a small wrinkle in the so far smooth Higgs boson picture. That small wrinkle that may point the way out of the SM oasis, across the desert, and into BSM physics. It's probably not a matter of "if" but a matter of "when", and therefore a matter of endurance and perseverance. Fifty years have passed since the seminal papers on the SM Higgs boson and today, and perhaps another 50 years may be needed to find what Nature has in stock beyond the Standard Model.
A more in-depth summary of these results is available in the CERN Courier.
The views expressed in CMS blogs are personal views of the author and do not necessarily represent official views of the CMS collaboration.